by Elvis Pillinen
Since the invention of the first laser in the 1960s, researchers have discovered that by mode-locking, optical pulses can be made much shorter, even down to mere femtoseconds. In human perspective, pulse created by a flashlight (millisecond, 10-3 s) is 300 km long, enough to stretch from Helsinki to Petersburg, and by comparison ultrashort pulses (femtosecond, 10-15 s) can be shorter than the width of human hair. Thousands of pulses are sent every second, enough to play sound, and after amplification this pulse train may contain as much energy as an airgun concentrated onto small area, not only easily destroying optical components (solution to which was awarded 2018 Nobel prize in physics) but also allowing nonlinear behavior from materials. Also, ultrashort pulse may even appear as white unlike more common continuous laser. In short, no pun intended, ultrashort pulses offer several important properties: they are extremely short, have high repetition rate and energy, and wide spectrum. These can be exploited in several ways. Applications of ultrashort pulses are found in classical and quantum computation as well as communication, metrology, nano optics, femtochemistry, etc.
Important motivation for my research comes from femtochemistry. The shorter the optical pulse the faster the phenomena that can be studied and controlled with it. Atoms move about 1 km/s; hence a fast “flashlight” is required to stop their motion. Initially in flash photolysis a modified flashlight was used, which was enough to study radicals between chemical reactions (Nobel in chemistry 1967) which later evolved into femtochemistry to study chemical reactions themselves directly (Nobel in chemistry 1999), further expanding understanding of things like photosynthesis, for example. It was also recognized that not only can pulses be used to study chemical reactions and atomic phenomena, but they can also be controlled to obtain a desired outcome. Such reactions include for example dissociation, isomerization, absorption, ionization, conversion between enantiomers, lattice vibrations, Raman scattering, coherent control, and many others. For these purposes, ultrashort pulse properties must be controlled in femtosecond scale. Initially researchers were able to control intensity and phase. However, atoms and molecules are quantum systems and hence are vectorial, so ideally polarization state should also be controlled. Ultrashort polarization modulation is a new research area which was experimentally demonstrated in the early 2000s offering wider control, better efficiency, and potentially new applications.
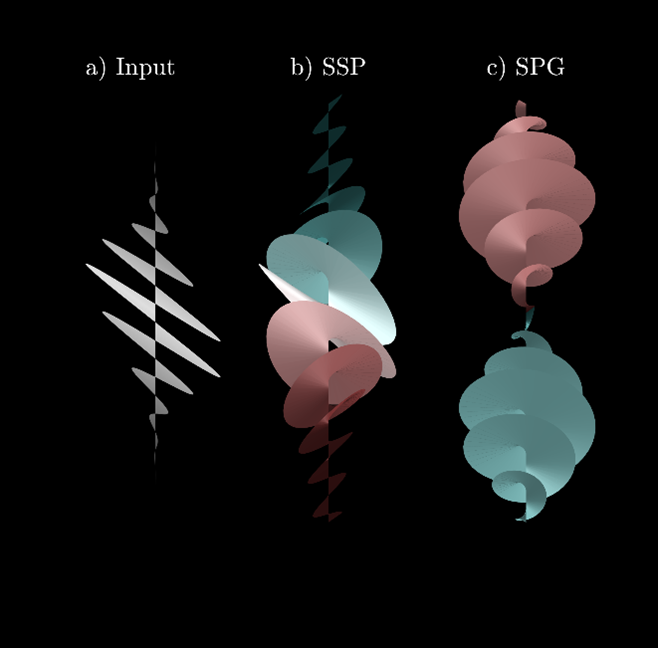
How does one control ultrashort pulses? They are so short that no semiconductor can control them directly, and approaches with nonlinear materials are fundamentally limited. Fortunately, spectral and temporal domains of light are linked to each other, the pulse defines the spectrum and the spectrum defines the pulse. Traditionally, pulses have been controlled by spectral modulation of phase or intensity, which allows to control pulse shape, phase, and coherence properties even in femtosecond scale. Spectral polarization modulation on the other hand differs by modulating intensity and phase in two directions simultaneously which allows to control polarization state of the pulses as well. Many of these polarization changes are cyclical, in my thesis and current research as well, and as such could be interpreted through the geometric phase which needs further study.
Most research of ultrashort pulses assumes a fully coherent and polarized pulse, but is this really the case? While often this is a valid approximation, in real life there is always a degree of randomness and light is then described as partially coherent (and partially polarized). This can happen naturally, even on propagation through air, or by design, for example supercontinuum laser. One of the effects of partial coherence is that such pulses are longer, but consequences are more profound as it affects how the pulse interferes. These random phenomena of light must be understood as they affect not only spectral modulation schemes, but also their applications in chemistry and others. Afterall, if such random pulse interacts with an atom, then said atom will also be randomly affected. Therefore, my thesis also theoretically considers spectral polarization modulation in the partially coherent case. Further research could study the effects in practical cases.
My thesis focused on two different modulators, spectral step polarizer (SSP) and spectral polarization gratings (SPG). Polarization gratings (PG) were initially examined in the early 2000s in the spatial domain. Since then, pulses created by SPG have been experimentally verified and applications have been proposed, for example in multidimensional spectroscopy. Last year we published a theoretical article on SPGs in the coherent case and we will publish an article also on the partially coherent case, as already presented in my thesis. SPGs have two main properties: high efficiency and separation of polarization states. They modulate the polarization state along the spectrum cyclically and the input pulse and its polarization components are split efficiently into several temporal copies. Compared to conventional scalar gratings which only control phase or intensity, SPGs can theoretically reach 100% efficiency. However, realistically the design must be discrete, limiting efficiency along with other practical issues. Also, the pulses can be overlapped to create flat shaped pulse design. Then the other example, SSP, which uses two different filters for both sides of the spectrum. This results in a rotating polarization state along the pulse and widened intensity distribution. In Fig. 1 we can see an artistic representation of pulses with a) 45-degree linearly polarized input pulse that is modulated by b) SSP and c) SPG duplicator. In the partially coherent case, an SPG acts similarly but the pulses are wider and intensities between pulses are altered. Interestingly, SSPs can demonstrate a link between partial coherence of the input and partial polarization of the output. For further details refer to my thesis where I have provided extremely detailed instructions for the theory and simulations, as well as some practical considerations for the partially coherent case.
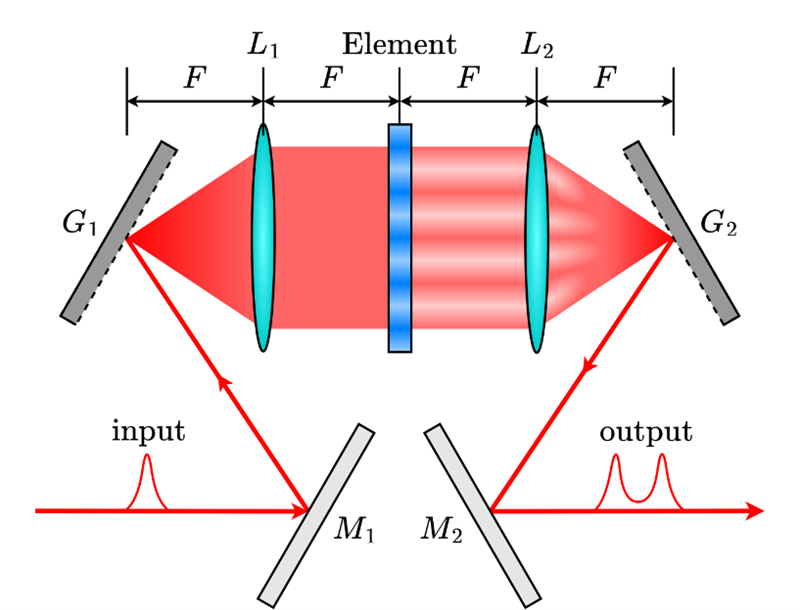
In practice, spectral polarization modulation can be realized with a common 4F-setup as illustrated in Fig. 2, where the pulse is transformed from temporal to spectral domain (and in reverse) by gratings and lenses and the spectral polarization is modulated by an optical element which produces an output pulse that we desire. Pulse characterization can be done with already well-established frequency resolved optical gating (FROG). Modulation can be done with passive elements or actively controlled for arbitrary modulation, but the latter is harder to accomplish. Partially coherent case is difficult to create and measure and more research is required. Due to equipment failure, my master thesis could not include measurements and instead only considered practical aspects of generation, modulation, and measurement of ultrashort pulses. Fortunately, we have recently acquired a new femtosecond laser and we are in the process of fabricating SPG elements for practical implementation. Science is like a tree, and multiple interconnected veins converge into my thesis, ultrashort lasers, diffraction gratings, partial coherence, and spectral modulation. Important justification for my thesis is found from the study and control of chemical and atomic reactions as well as from other real-world applications. My thesis consists mainly of theoretical examination of spectral polarization modulation and as examples I considered SSP and SPG elements with several interesting properties. My work also offers an opportunity for further research such as the case of partially coherent pulses, as well as examining the polarization state and the geometric phase in the quantum optical framework. More research is required to fully understand partially coherent pulses and their effects.