Jonas Suni, University of Helsinki
Space physics concerns the study of the near-Earth plasma environment, which stretches from around 60 kilometres to hundreds of thousands of kilometres above the Earth’s surface. This vast volume of space can however be divided into a few different regions of interest.
Closest to the Earth is the ionosphere, which consists of partially ionised gases from the Earth’s atmosphere. This is where aurora form, due to the precipitation of energetic particles from the magnetosphere. The magnetosphere sits above the ionosphere, and it consists of plasma mostly trapped in the Earth’s magnetic field. Outside of the magnetosphere, the majority of the plasma comes from the Sun in the form of a continuous high-speed stream of plasma known as the solar wind, which is magnetised by the interplanetary magnetic field (IMF) that originates in the Sun. The magnetosphere acts as a flow obstacle for the incoming solar wind, and a bow shock forms ahead of the magnetosphere because the solar wind is faster than the fastest local signal speed. At the bow shock, the solar wind is slowed down, compressed, and heated as it begins to flow around the magnetosphere, forming a region known as the magnetosheath. The boundary between magnetosheath and magnetosphere is called the magnetopause. On the solar wind side of the quasi-parallel bow shock, i.e. where the shock normal is close to parallel to the IMF direction, solar wind particles reflected from the bow shock cause the formation of a foreshock.
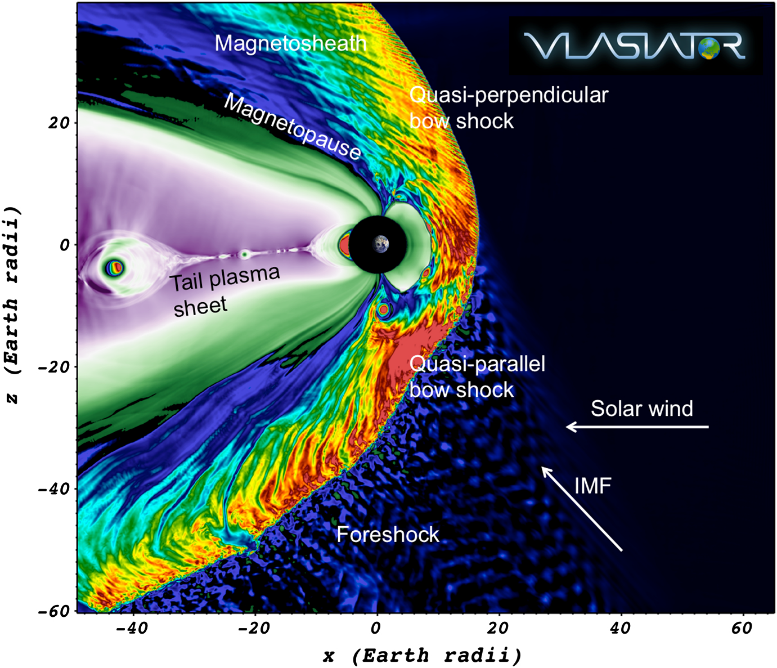
All these regions and boundaries are host to a variety of interesting plasma and electromagnetic phenomena. My research focuses on a phenomenon called magnetosheath jets, which as the name suggests occur in the magnetosheath. There are myriad definitions for what constitutes one of these jets, but most define them as transient pulses of high dynamic pressure (mass density multiplied by velocity squared) in the magnetosheath. Jets usually have durations on the order of tens of seconds and can be larger than the Earth. If an earthward-directed jet lasts long enough, it can impact the magnetopause, disturbing the magnetosphere shape, transferring energy and mass and launching waves into the magnetosphere. This can expose satellites and other spacecraft to increased fluxes of energetic particles and contribute to accumulation of charges that can damage electronics. In addition, jets have been associated with disturbances in ground-based magnetometer measurements, which indicates the presence of ionospheric currents. These currents can induce currents at ground level as well, which can damage power network infrastructure.
Jets have been observed by spacecraft for over two decades now, but they remain shrouded in mystery. This is partially because spacecraft can only be in one place at one time, and thus they are of limited use in studying transient phenomena like jets. This is where simulations come in. Global simulations model the entire magnetosphere, and they are limited only by their spatial and temporal resolutions, as well as the accuracy of their physics. Vlasiator is a global hybrid-Vlasov simulation, where ions are modelled as distribution functions in 3D real space and 3D velocity space that obey the Vlasov equation (the collisionless Boltzmann equation under the Lorentz force), electrons are modelled as a charge-neutralising fluid, and the electromagnetic fields obey Maxwell’s equations and Ohm’s law. This model has been shown to accurately reproduce satellite observations in multiple regions of near-Earth space.
In my thesis, I used Vlasiator 2D (one simulation cell thick in one direction) simulation runs to study jet formation, which is difficult to do with spacecraft data. It is not impossible, however, to develop theories that are supported by spacecraft data. One of the earliest prominent theories is that waves in the foreshock upstream of the quasi-parallel bow shock cause the shock surface to ripple, letting solar wind plasma flow through the shock without slowing down significantly. Another theory is that the foreshock waves steepen to form structures of enhanced magnetic field (called SLAMS) that are transmitted through the bow shock to form jets. For my thesis, I developed an algorithm that identifies individual jets in Vlasiator simulation data and tracks them over time. The algorithm also identified and tracked what I call Foreshock Compressive Structures (FCS), which are defined as regions of enhanced magnetic field strength and dynamic pressure upstream of the bow shock. I then conducted a statistical analysis of the jets and FCSs, which revealed that up to 75% of jets that form at the bow shock are associated with FCSs impacting the bow shock.
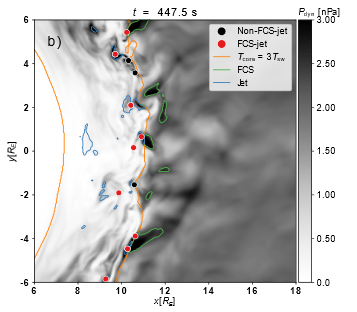
This result supports the SLAMS theory of jet formation. Interestingly, we also found evidence of bow shock ripples, but rather than existing as an alternative to the FCSs impacting the shock, it appears that the ripples are a consequence of the interaction between FCSs and the bow shock. The origin of the remaining 25% of jets, the ones that aren’t associated with FCSs, however, remains unknown.
Answering this outstanding question, as well as many other fascinating questions concerning the properties and effects of magnetosheath jets in different 2D and 3D Vlasiator simulations, will be the focus of my university grant-funded doctoral research project over the next 4 years. Ultimately, I’m hoping to be able to shed some light on how jets can affect the transfer and distribution of energy in the dayside magnetosphere, as this has tangible in the form of possible damage to spacecraft and ground-based infrastructure. There are still plenty of things to discover and learn about jets, and I am excited to be a part of this process.